From blending spirits for the most exquisite cocktails to intricately mixing lipids and nucleic acids for life-saving lipid nanoparticles (LNPs), the often unnoticed combination of two solutions can play a major role in our everyday lives. In the context of nucleic acids-LNPs, the most basic formulation requires the introduction of two separate feed solutions: one aqueous solution that includes the nucleic acid payload and one ethanolic stock with lipid components at the desired molar ratios. Laboratories from around the world have devised ingenious methods and geometries to mix these solutions together, producing highly efficacious LNP therapeutics. At their basic core, two major types of mixing are used today for the manufacturing of LNPs: turbulent and microfluidic mixing.

Figure 1.
The type of mixing has an influence on the ideal process parameters, design considerations, laboratory footprint, and scalability in the manufacturing of these particles. In this article, we at Helix Biotech, Inc. will delve into the two major types of mixing used to manufacture LNPs and discuss their implications on upscaling downstream manufacturing processes.
What is Microfluidic and Turbulent Mixing?
Microfluidic Mixing
Microfluidic mixing, as its name suggests, employs microscale chips with channels and chambers on the micrometer length-scales. Fluid lines are then connected to these microchannels, where the fluids are directed, mixed, separated, and can even be analyzed within a single chip. At such small scales, microfluidics require low sample volumes (µL range) as well as low amounts of chemicals and raw materials, reducing global costs per experiment. Microfluidic chips themselves can also be small and compact, which reduces their laboratory footprint.
Because of its continuous laminar flow, microfluidic mixing typically relies on diffusion between the adjoining streams. This process is inherently slower than turbulent mixing but can be improved by using different geometries to introduce some turbulence or by incorporating longer channels for greater mixing times. Some of the most commonly used and commercialized configurations can be seen in Figure 2 (left). Of note, microfluidic mixing successfully played a role in the development of Onpattro®, the first FDA-approved siRNA-LNP therapeutic[1].

Figure 2. Various geometries and configurations of microfluidic (left) and turbulent (right) mixers employed today.
Turbulent Mixing
On the other hand, turbulent mixing occurs when liquids at high flow rates collide and induce turbulent flow patterns, shearing forces, eddies, and vortices. This process is characterized by chaotic and random interactions for rapid and thorough mixing to produce homogeneous solutions. Think about the mixing of milk in coffee where stirring induces turbulence and promotes mixing. In terms of nanoparticles (NP), turbulent mixing typically occurs in small chambers that come in numerous geometries, e.g. coaxial turbulent jet mixers, impingement jet mixers (IJM) and multi-inlet vortex mixers (MIVM) as seen in Figure 2 (right), that rapidly mix multiple liquid inputs[2]. In impinging jet mixers, mixing is achieved through the collision of fluid jets at an impingement point; in MIVM, mixing occurs through the swirling motion (i.e. vortex) created in the central chamber.
Now, one of the premier advantages of turbulent mixing is in the speed of fluid mixing prior to nanoparticle formation. This mixing ensures complete homogeneity of the two fluids containing building blocks for the nanoparticles, prior to the initiation of nanoprecipitation which then produces uniform NP formation within milliseconds. If this process is too slow, the resulting NPs may have greater size and polydispersity, and lower mRNA encapsulation efficiency. Coupled with their ability to harness fast fluid flow rates for small and large-scale production at comparable process footprints, turbulent mixing is a very attractive alternative to microfluidic mixing–so attractive that the COVID-19 vaccine manufactured by Pfizer-BioNTech (Comirnaty) employs turbulent mixing to produce billions of vaccine doses[3].
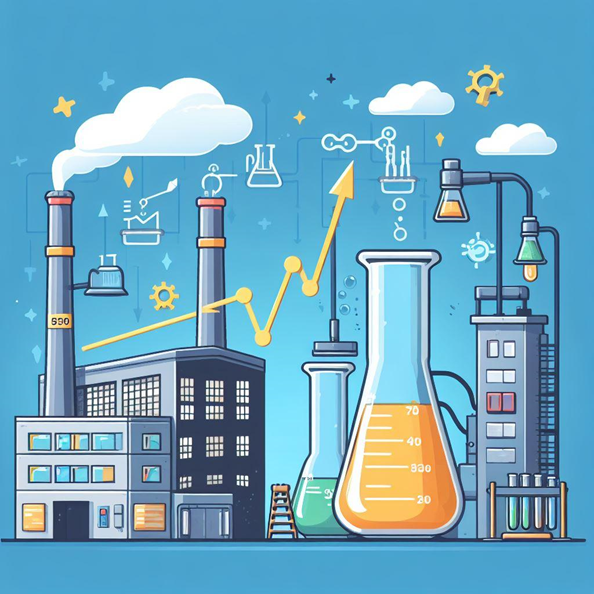
Figure 3.
Scaling Up and Not “Scaling Out”
Microfluidic Mixing
One of the fundamental challenges in getting any drug product to market is transitioning from small-scale R&D laboratory preps into large-scale commercial production. Microfluidic systems are characterized by microscale channels; while this is advantageous for precise and controlled experimentation, the inherent limitation is the low throughput. The production volume achievable is often orders of magnitude smaller than what is required for commercial drug manufacturing. Increasing flow rates often leads to channel clogging. Two strategies to enhance throughput is to employ continuous flow in a single chip, redesign of the chip to accommodate higher flow rates, or to design parallelized microfluidic systems or array configurations[4]. The latter involves running multiple microfluidic devices simultaneously to increase the overall production rate. However, this introduces engineering and logistical challenges in coordinating the operation of multiple devices and ensuring uniformity across all channels–all of which heightens the added capital costs[5].
The commercially available microfluidic mixers often employ single-use microfluidic cartridges which minimizes the technical requirements needed to produce LNPs. Nevertheless, even when dismissing potential environmental concerns, one must be wary of the potential for large recurring costs due to their single-use nature, cartridge-to-cartridge variation, and solvent-cartridge compatibility[6]. Thus, with microfluidic systems, scaling up instead of “scaling out” requires a delicate balance between increasing output while maintaining the precise and careful mixing within the chip.
Turbulent Mixing
With regards to turbulent mixing, it is a dynamic and efficient process that has shown substantial promise in large-scale production of LNPs, as exemplified by the Pfizer-BioNTech COVID-19 vaccine. The beauty in the nanoprecipitation process enabled by turbulent mixing is that with a minimal footprint, hundreds of milligrams of nanoparticles can be produced per minute [7]. Meanwhile, microfluidics at the R&D stage typically have production rates below 1 mg/min and only with special configurations can it slightly increase the throughput[7].
There are key considerations that need to be explored when upscaling. The ideal turbulent conditions must be maintained within the mixing chamber when reaching higher flow rates. An important concept to introduce here is the Reynolds number (Re, Re = ρVD/μ, ρ - density, V - velocity, D - characteristic length, μ - dynamic viscosity) which predicts a flow’s nature. Higher Re indicates increased turbulence, whereas lower Re is associated with a higher likelihood of laminar flow. Microfluidic mixers typically mix solutions at an Re of less than 100, whereas turbulent mixers occur between 100 and 2000. Increasing the total flow rate (TFR, i.e. velocity in the Re equation) for higher throughput will lead to an increase in Re for a given mixing chamber. Very high Re (>4000) can cause excessive turbulence which can lead to sample inhomogeneity and CQAs. Luckily, the relation between Re and TFR is straightforward and can be used to predict the appropriate mixing chamber size to employ.
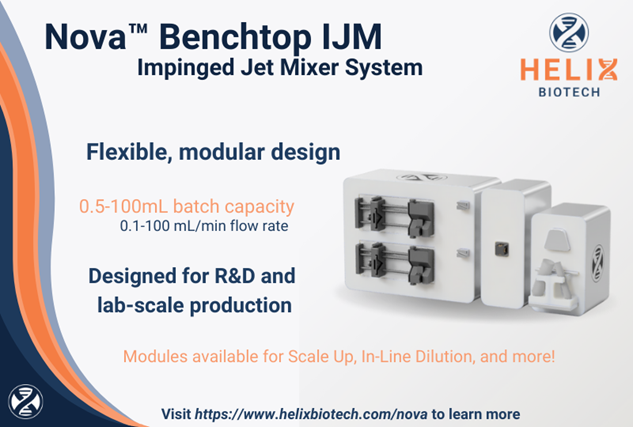
Figure 4. Nova™ Benchtop NP manufacturing system. The Nova™ is designed for high throughput screening of a multitude of nanoparticle compounds and therapeutics.
Concluding Remarks
Helix Biotech’s Nova™ Benchtop (BT) system is a NP manufacturing system which employs impinging jet mixers for rapid nanoprecipitation. Researchers can quickly screen and optimize lipid, polymer, or hybrid nanoparticle formulations, while maintaining a path for upscaling in the future. The Nova™ Platform is a modular system, meaning scaling up is as easy as adding modules containing continuous flow pumps and/or mixer modules for in-line dilutions. It is microfluidic chip compatible, but the Nova™ IJM mixers can be reused many times through simple cleaning steps, reducing global costs when compared to consumable-based chips. In the end, all Nova™ BT processes and mixing technologies are designed to facilitate a seamless technology transfer and process scale-up when commercial production is required.
When deliberating between turbulent and microfluidic mixing for Lipid Nanoparticle (LNP) production, both methods showcase the ability to produce monodisperse LNPs on the nanometer length-scales, each with its unique advantages. Microfluidic mixing offers a precise and controlled environment for research and development of LNPs. In contrast, turbulent mixing offers a robust and proven solution that is well-suited for the whole drug development pathway from pre-clinical research all the way to commercial manufacturing. By carefully weighing the factors mentioned here, researchers and manufacturers can make informed choices that align with the specific requirements of their intended end goal, paving the way for advancements in the field of nanomedicine.
References
Kulkarni, J. A., Witzigmann, D., Chen, S., Cullis, P. R., & van der Meel, R. (2019). Lipid Nanoparticle Technology for Clinical Translation of siRNA Therapeutics. Accounts of Chemical Research, 52(9), 2435–2444. https://doi.org/10.1021/acs.accounts.9b00368
Shepherd, S. J., Issadore, D., & Mitchell, M. J. (2021). Microfluidic formulation of nanoparticles for biomedical applications. Biomaterials, 274, 120826. https://doi.org/10.1016/j.biomaterials.2021.120826
Warne, N., Ruesch, M., Siwik, P., Mensah, P., Ludwig, J., Hripcsak, M., Godavarti, R., Prigodich, A., & Dolsten, M. (2023). Delivering 3 billion doses of Comirnaty in 2021. Nature Biotechnology. https://doi.org/10.1038/s41587-022-01643-1
Huang, Y., Liu, C., Feng, Q., & Sun, J. (2023). Microfluidic synthesis of nanomaterials for biomedical applications. Nanoscale Horizons. https://doi.org/10.1039/D3NH00217A
Valencia, P. M., Farokhzad, O. C., Karnik, R., & Langer, R. (2012). Microfluidic technologies for accelerating the clinical translation of nanoparticles. Nature Nanotechnology, 7(10), 623–629. https://doi.org/10.1038/nnano.2012.168
O’Brien Laramy, M. N., Costa, A. P., Cebrero, Y. M., Joseph, J., Sarode, A., Zang, N., Kim, L. J., Hofmann, K., Wang, S., Goyon, A., Koenig, S. G., Hammel, M., & Hura, G. L. (2023). Process Robustness in Lipid Nanoparticle Production: A Comparison of Microfluidic and Turbulent Jet Mixing. Molecular Pharmaceutics, 20(8), 4285–4296. https://doi.org/10.1021/acs.molpharmaceut.3c00390
Valencia, P. M., Farokhzad, O. C., Karnik, R., & Langer, R. (2012). Microfluidic technologies for accelerating the clinical translation of nanoparticles. Nature Nanotechnology, 7(10), 623–629. https://doi.org/10.1038/nnano.2012.168